Research Themes
Our research focuses on cofactor-driven biocatalyses that contribute to human health or address a biotechnological need.
Understanding biological iron and heme
Iron is the most abundant transition metal in the earth’s crust and an essential element
for nearly every form of life. Iron’s unique place in biology is due to its ability
to shuttle electrons at biologically attainable redox potentials. This put iron at
the center of critical processes from respiration to dioxygen trafficking and a host
of carbon conversion reactions. When acting as a catalyst, biological iron can bind
directly to amino acid side chains, alone or as part of inorganic clusters with sulfide
or other ligands. Or, iron can bind at the center of heme. Most of the ~3 grams of iron in the human body
exists as part of a heme cofactor: the complex of Fe(II) with the protoporphyrin IX
(PPIX) macrocycle. This complex is similar in structure to other biological metallocycles,
including vitamin B12 (cobalamin), chlorophyll (magnesium-bound PPIX), and the Ni(II)
complex known as F430, which is essential for the biosynthesis of methane. These beautifully
colored cofactors collectively control some of the most biologically and ecologically
significant processes on earth.
Discovering how the microbiome contributes to human nutritional iron and protects against disease
The human microbiome is made up of thousands of different species. The majority of the organisms in the gastrointestinal microbiome are anaerobic species that cannot make heme, even though they require it for their own survival. At the same time, there is no well described heme transporter in the cells lining the human gastrointestinal tract. Mismanagement of heme or iron in the GI tract leads to a range of diseases, including inflammation and cancers. The microbiome has been proposed to facilitate the uptake of iron by the host and to serve as a buffer against disease.
How do members of the microbiome recruit the heme and iron they need? How do their metabolic activities impact the host? Our current work aims at identifying and describing the molecules and metabolic pathways these organisms use to metabolize iron, support host nutrition, and prevent diseases associated with redox stress.
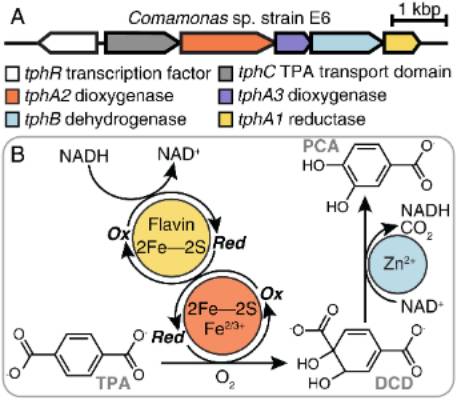
Three step enzymatic pathway for converting a plastic monomer into a useful metabolite.
Microbial reactions that transform waste into useful materials
Oxidations are a large class of reactions with vital applications in biotechnology and medicine. Dioxygen is nature’s favorite chemically “green” oxidant: abundant, thermodynamically powerful, and, when properly, controlled, non-toxic. Typically, biological reactions begin by activating dioxygen using a protein-bound iron, heme, or flavin cofactor.
We are studying how these catalysts work and investigating applications of these and other microbially mediated reactions to pressing environmental problems. The goal of this work is to use renewable strategies to carry out important bulk reactions, particularly the conversion of biological polymers and plastics to useful products.
Carboxylases that convert atmospheric carbon dioxide to biomass.
The most abundant enzyme on Earth, Rubisco, catalyzes the first step in the fixation
of carbon dioxide into biomass in green plants. We now know, however, that this is
one of many processes in the biosphere in which carbon dioxide is used as a carbon
source. We are currently studying three microbial mechanisms for the fixation of carbon
dioxide or its hydrated counterpart, bicarbonate. Our work in this area aims at developing
an understanding of the many catalytic strategies by which nature makes use of carbon
dioxide.
Acetone carboxylase uses manganese to condense carbon dioxide from air and acetone to make acetoacetate – a building block for biomass.